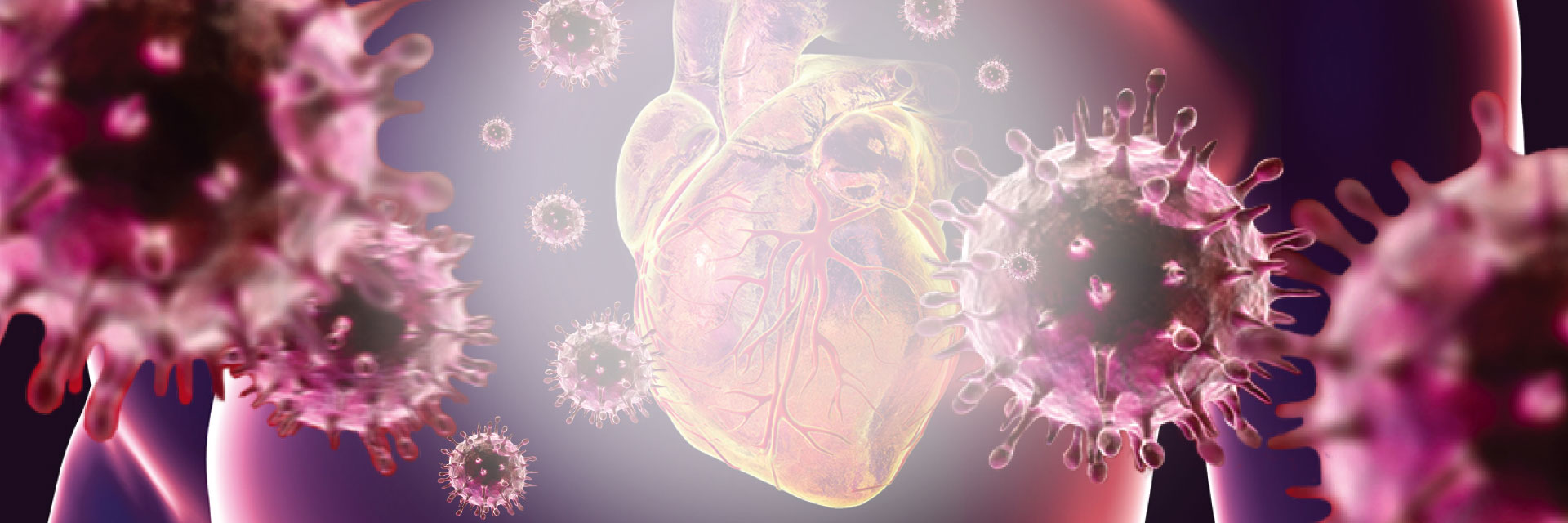
Engineered Immortals that Package a Potent Punch
Cell therapy using cardiosphere-derived cells (CDCs) is an increasingly promising treatment for acute and congenital heart muscle injuries, including myocardial infarction (MI). The therapeutic potency of CDCs has been attributed to the cargo they secrete via exosomes and other extracellular vesicles (EVs), which act to suppress inflammatory and fibrotic processes, whilst promoting cell renewal. Unfortunately, broad clinical application of CDCs and/or their EVs is obstructed by the variability of CDC potency among wild-type populations from healthy donors. Furthermore, cultures of potent donor isolates inexplicably demonstrate reduced efficacy from lot to lot, and immortalized CDCs (imCDCs) lack potency altogether. In order to predict, manipulate, and maximize the therapeutic efficacy of CDCs and their EVs for medical applications, elucidation of the mechanisms underlying their potency is crucial.
The recently published work of Ahmed G. E. Ibrahim and colleagues, headed by L.A.-based doctors Linda and Eduardo Marbán, offers key insights into this process. Published in Nature Biomedical Engineering, ‘Augmenting Canonical Wnt Signaling in Therapeutically Inert Cells Converts Them into Therapeutically Potent Exosome Factories’ (summarized by Figure 1) has identified β-catenin as a biomarker and central mediator of CDC potency. Their findings enable identification of effective CDC and EV populations, whilst pinpointing a means to drive the transformation of low potency and non-therapeutic CDCs into highly potent therapeutic agents.
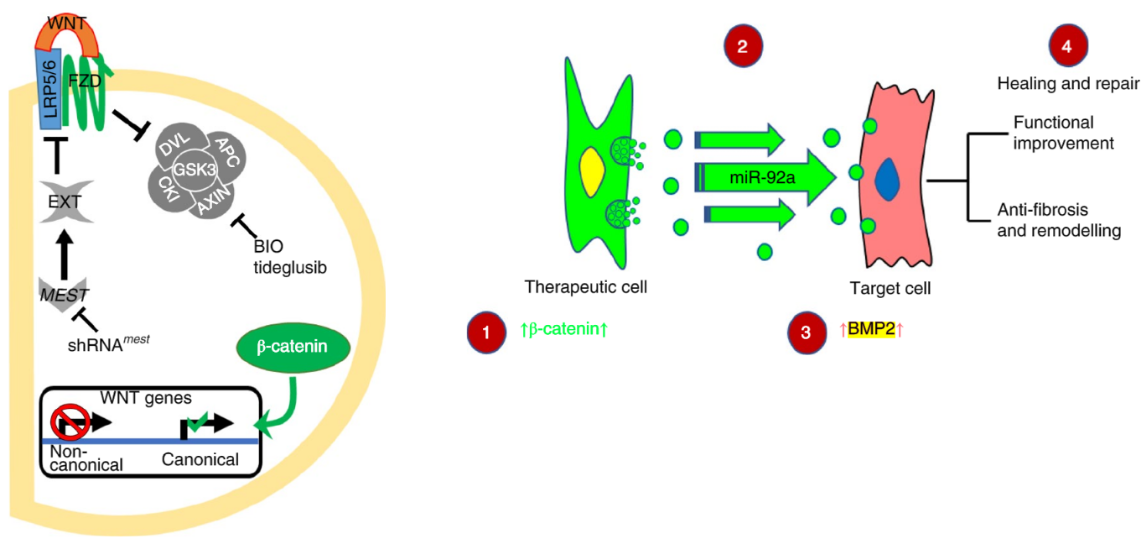
Figure 1*: β-catenin activation in CDCs leads to enrichment of miR-92a in secreted EVs. β-catenin signaling (1) alters CDC EV contents including enrichment of miR-92a (2). Secreted EVs are uptaken by target cells and activate BMP2 signaling (3), leading to healing and repair (4).
Through transcriptomic, proteomic, and histological analyses, it was found that high-potency CDCs expressed β-catenin at levels approximately double that of low-potency CDCs via the canonical Wnt/β-catenin signaling pathway, as opposed to non-canonical pathways predominant in low-potency populations. Therapeutic efficacy of low-potency CDCs could then be enhanced by increasing the abundance of β-catenin via inhibition of proteosomal degradation using 6-Bromoindirubin-3′-oxime (BIO). Through the same mechanism, low-potency cell lots from otherwise high-potency CDC banks were ‘corrected’ when treated with BIO. Even imCDCs—which are virtually non-potent—were morphed into high-potency CDCs following β-catenin augmentation with BIO; however, consistent stabilization for clinical purposes proved difficult.
Hand-in-hand with increased β-catenin abundance in high-potency CDCs, qPCR and RNA sequencing revealed significant downregulation of Mesoderm-specific Transcript (Mest), as well as microRNA miR-335, in BIO-treated CDC populations (Figure 2 A)—both of which are post-transcriptional inhibitors of low-density lipoprotein receptor 5/6 (LRP5/6), which is involved in the prevention of β-catenin degradation. Interestingly, decreased Mest following β-catenin overexpression was not observed in inert non-cardiac cells. However, when such cells were treated with EVs derived from high-potency CDCs, significantly decreased Mest was observed, supporting hypotheses of EV-mediated CDC efficacy.
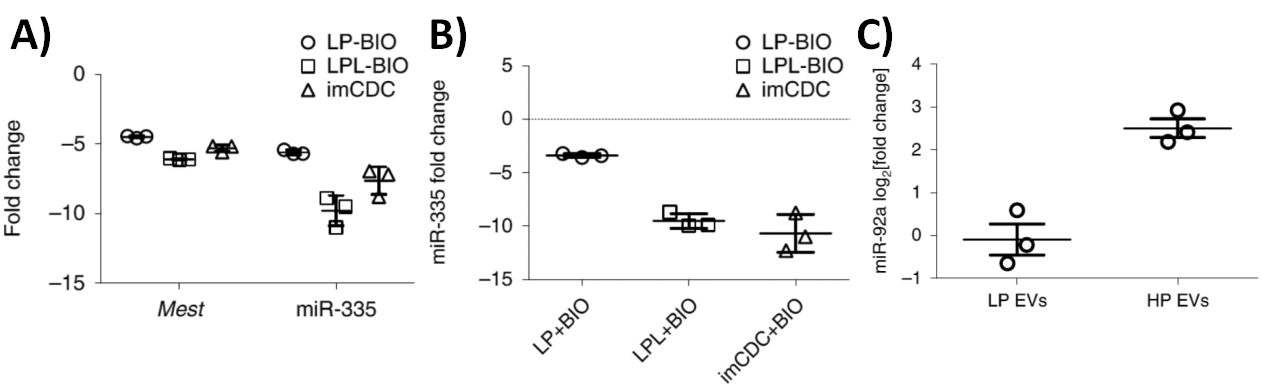
Figure 2*: A) qPCR validation of Mest and miR-335 changes in LP (low-potency), LPL (low-potency lots) and imCDCs exposed to BIO, normalized to DMSO. B) Gene expression fold change of miR-335 in EVs isolated from BIO-treated LP, LPL, and imCDCs compared with their vehicle control counterparts (n = 3; mean ± SD). C) Enrichment of miR-92a in HP (high-potency) EVs compared with LP EVs (n = 3; mean ± SEM; P < 0.001).
NORBLOG
Want to hear more from Norgen?
Join over 10,000 scientists, bioinformaticians, and researchers who receive our exclusive deals, industry updates, and more, directly to their inbox.
For a limited time, subscribe and SAVE 10% on your next purchase!
SIGN UP
Indeed, exosomal RNA gene expression analysis following exosomal RNA isolation using the Urine Exosome RNA Isolation Kit (Norgen Biotek Corp.) revealed that miR-335 was significantly reduced within the EVs of high-potency CDCs (Figure 2 B) with other key microRNAs remaining unchanged, demonstrating distinct modulatory EV cargo from β-catenin-enriched CDCs. Notably, EVs from all high-potency CDC populations were high in miR-92a (Figure 2 C), believed to activate the canonical Wnt/β-catenin pathway via Bmp2 stimulation (Figure 1). Armed with an arsenal of data demonstrating the key role of β-catenin and other mechanisms underlying CDC potency modulation, Ibrahim and colleagues sought to engineer immortal and highly potent EV factories from ordinary inert cells (normal human dermal fibroblast; NHDFs) as an alternative to problematic donor CDCs and imCDCs. Without augmentation, NHDF were detrimental to healing when injected into MI mice (Figure 3 A). However, mortality was comparatively improved when these mice were treated with NHDF which were overexpressing β-catenin, particularly in combination with cardio-protective Gata4 over-expression (NHDFβ-catenin/Gata4).
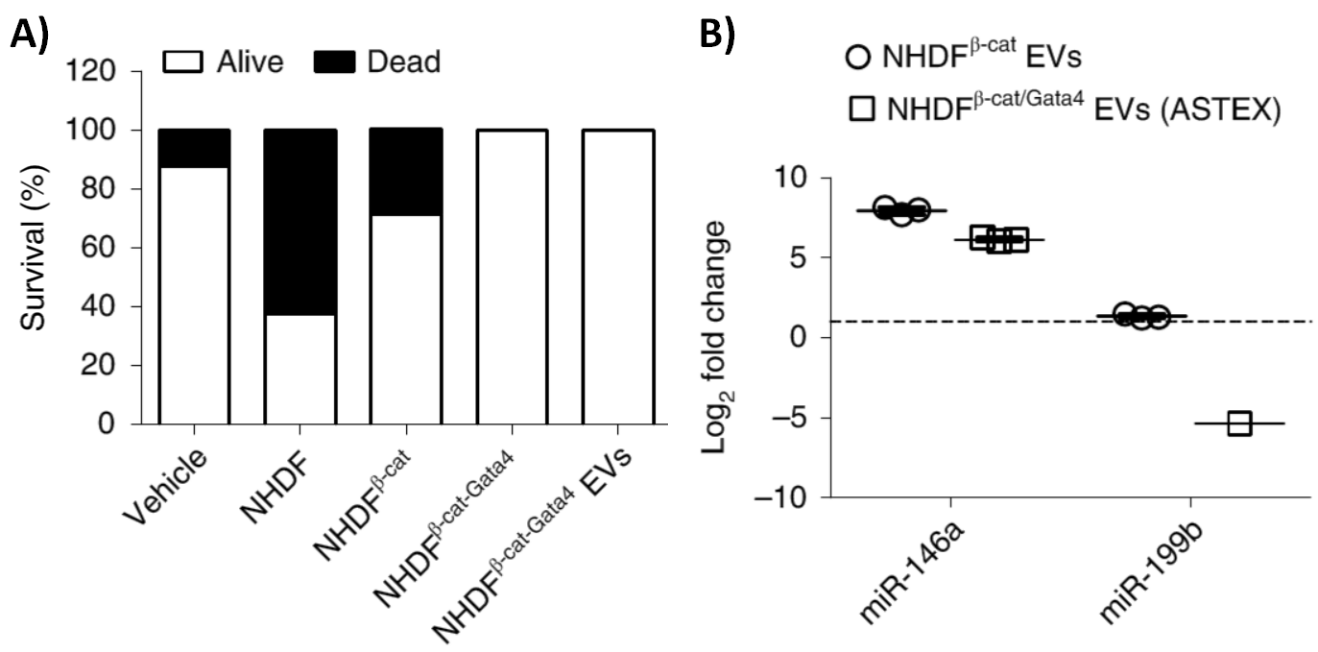
Figure 3*: A) Mortality of MI mice following cell and cell-free therapies. B) qPCR of microRNA markers in EVs of transduced cells (n = 3; results normalized to NHDF EVs).
As with other high-potency CDCs, it was found that NHDFβ-catenin/Gata4 cells were enriched with miR-92a as well as miR-146a—another species of therapeutic microRNA common to high-potency CDCs. However, unlike wild-type CDCs, NHDFβ-catenin/Gata4 exhibited reduced levels of miR199b—a negative regulation associated with pathological remodeling—as well as diminished senescence akin to immortalization, possibly due to synergistic roles of β-catenin and Gata4 in cell growth. These highly potent, immortal-like cells were termed Activated-Specialized Tissue Effector Cells (ASTECs). Not only was cell therapy successful with ASTECs, but MI mice that underwent cell-free therapy with EVs from ASTECs fared equally well (Figure 3 A). These highly potent ASTEC-derived EVs, termed ASTEX, were found to have similar microRNA profiles to ASTECs—enriched with miR-92a and miR-146a, but low in miR-199b (Figure 2 A; Figure 3 B), further pointing to EV-mediated therapeutic potency.
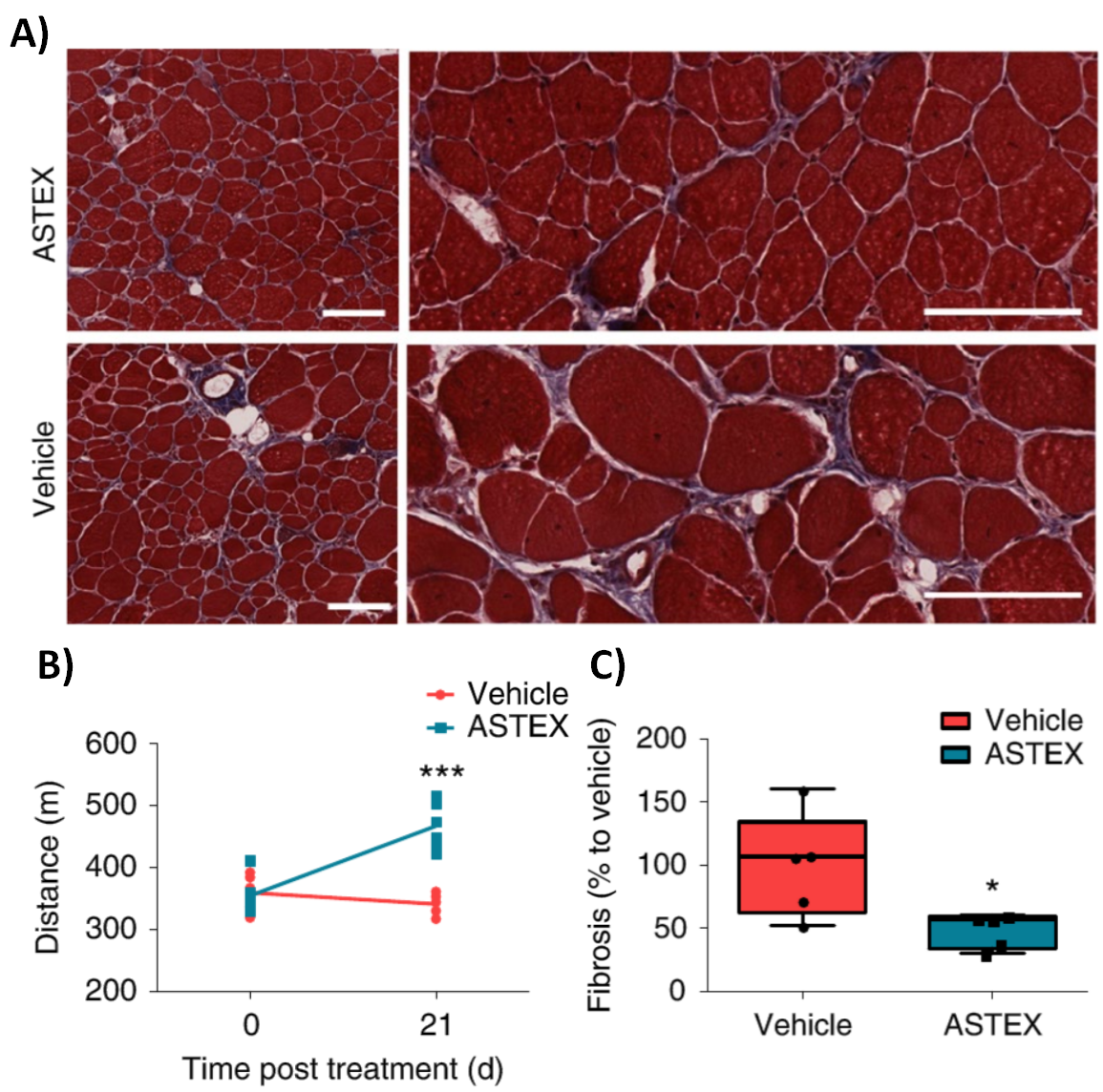
Figure 4*: A)Representative micrographs from vehicle and ASTEX-injected DMD mouse muscle. B) Maximal exercise capacity after 21 days (n = 5; mean ± s.e.m., P < 0.001). C) Muscle fibrosis (%) in DMD mouse muscle 3 weeks after ASTEX injection (n = 5; mean ± max. min. ; P = 0.015).
Isolated ASTEX were then used to treat skeletal muscle in Duchenne muscular dystrophy (DMD) mice, effectively demonstrating the utility of ASTEX for cell-free therapy. In less than one month following ASTEX therapy, treated DMD mice could run significantly faster (Figure 3 B) and displayed reduced fibrosis (Figure 3 A, C) compared to untreated DMD mice. This remarkable research, elucidating molecular mechanisms underlying CDC potency, ultimately enabled these scientists to transform ordinary skin cells into ASTEX warriors against heart injuries, with potential extending far beyond.
*Figures and figure legends adapted from IIbrahim, A.G., Li, C., Rogers, R., Fournier, M., Li, L., Vaturi, S.D., Antes, T., Sanchez, L., Akhmerov, A., Moseley, J.J. and Tobin, B. (2019). Augmenting canonical Wnt signalling in therapeutically inert cells converts them into therapeutically potent exosome factories. Nature biomedical engineering, 3(9), 695-705.